February 1958 Popular Electronics
Table
of Contents
Wax nostalgic about and learn from the history of early electronics. See articles
from
Popular Electronics,
published October 1954 - April 1985. All copyrights are hereby acknowledged.
|
If you have never paid
much attention to the state of the art in telescopes, then you might believe they
consist of either the $99 Tasco jobs in Walmart's toy department or the 200" Hale
atop Mount Palomar, with not much inâbetween. You might also think that objects which emit or reflect light
in the visible spectrum are the only things studied. You might not know that telescopes
have been built to detect frequencies ranging from HF all the way up into cosmic
rays. Newer telescopes search for gravity waves and even neutrino and "dark matter"
pseudo particles. You might not know that there is a broad selection of optical
telescopes for amateur and research grade telescopes (Celestron 8SE is a great mid-range telescope) and that the line between amateur
and research grade telescopes grows less distinct every year. Even radio telescope
equipment is beginning to hit the market. This article gives a nice intro to the
subject of radio telescopy; the basics haven't changed.
Other related articles:
"How We Listen to Stars and Satellites" - January 1958 Popular
Electronics, "Radio Astronomy and the Jodrell Bank Radio Telescope" - February
1958 Radio & TV News, "Radio Astronomy - Low Noise Front-Ends" - June 1954 Radio &
Television News, "Radar
Explores the Moon" - May 1961 Popular Electronics, "Cosmic Radio Signals from Sun and Stars" - March 1948 Radio-Craft
How We Listen to Stars and Satellites

Long-range radar is used to track the Sputniks in their orbits.
A typical transmission-response pattern appears above the symbol for the International
Geophysical Year.
Radio and radar help astronomers search outer space
By Mike Bienstock, Associate Editor
It wasn't very long ago that astronomy, like the mythical Cyclops, had only one
eye - the optical telescope. Astronomers expected that bigger and better giant "eyes"
would unlock the remaining closed doors of the universe. Everything, it seemed,
was progressing quietly in its well-ordered way.
Then a second eye was developed, that of radio astronomy. A whole new universe
opened - an incredible dimension they didn't know existed. Today radio astronomy
is flourishing like a lusty youngster; it may some day equal or even exceed in importance
the 4000-year-old science of visual astronomy.
Another branch of the science is long-distance radar, which is now coming into
its own in tracking the Russian Sputniks. As a matter of fact, some of the giant
radio telescopes have had radar antennas installed in them for this job.
Static Identified. Radio astronomy is the science dealing with radio-frequency
emissions from the stars, a phenomenon never suspected until about 1930, when Karl
Jansky, a Bell Laboratories engineer, began to study and measure different kinds
of static at frequencies around 20 mc. Before long he realized that the hiss-type
static which he encountered was being emitted from definite points in space. Working
in his own backyard observatory, Grote Reber, another radio engineer and a radio
amateur, confirmed Jansky's discovery. Using a small parabolic antenna, he plotted
the first radio star sky map.
Giant strides have been made from these small beginnings. Dozens of radio telescopes
are scattered across the earth, their sensitive antennas constantly probing the
heavens, recording the strange radio impulses. We now know that three types of "stars"
emit radio waves: huge hydrogen gas clouds, made up of such a thin diffusion of
atoms that they would be called vacuums on earth; novae, which are stars that have
exploded with awesome violence; and collisions of huge star clusters or universes
called galaxies.
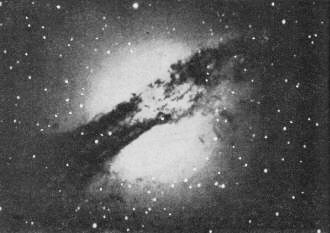
Astronomers call this probable radio source "NGC 5128" - it is
thought to be two galaxies in collision. This photo was made with the 200-inch Palomar
optical telescope.

Scientist measures and records radio observations of satellites
at Lincoln Lab's new long-range radar station, Lexington, Massachusetts.
Heretofore, one of the stumbling blocks for astronomers had been the huge masses
of dust scattered about the galaxies which prevented light from more distant stars
from being seen on earth. Scientists could only guess what lay beyond them. Now,
however, radio astronomy is unlocking even this secret. By focusing on gas cloud
signals coming from behind the dust pockets (at a frequency of 1420 mc.) , astronomers
have been able to "count" the number of stars beyond. Mind you, they are not only
counting the number of gas clouds whose radio emissions pass easily through the
dust but - mathematically - the actual number of "visual" stars. They have learned
that the greater the radio emission from an area, the greater the number of stars
that are located there.
Sources of Emission
Radio emission from gas clouds was first predicted in 1944. It wasn't until 1955,
however, that the signal was picked up on radioscopes. But since then astronomers
have made up for lost time. They have used the 1420-mc. signal, and shifts in this
frequency caused by the Doppler effect, to determine how fast and in which direction
the gas clouds are moving. Such data have allowed them to plot the movement of our
own galaxy, the Milky Way, as well as to gather more information on our expanding
universe.
The second most intense radio source, in the constellation of Cygnus, has been
found to be from two whole galaxies in collision about 200 light years away. Another
is listed only as NGC 5128 by astronomers. Others are being charted.
Our own sun has been proving a fruitful source of radio propagation. Although
the study of the radio spectrum of the sun was begun only within the past few years,
it has been determined that a huge amount of radio energy comes from the areas around
large and active sunspots. This is in the 5-meter band. It is thought that the flares,
being highly ionized gas, may produce strong electric fields when given rotational
motion, which in turn may be the cause of the radio emissions.
Of the planets, Jupiter was the first to be picked up on radioscopes; the signals
are apparently due to large-scale atmospheric disturbances. Venus was next to be
detected. Radio-frequency measurements showed this planet to have a temperature
higher than that of boiling water. Optical measurements had shown only half that
temperature, but since Venus is covered by a layer of clouds, the optical measurements
only took the cloud surface into account. Mercury, Mars and Saturn are expected
to be heard from soon via their radio signals. And some cosmic static comes from
"dark" areas, where stars have never been seen.
Two Types of Scopes
The radiotelescope is usually one of two types, the parabolic reflector (dish),
and the interferometer. There are other types - helical, horn, and combinations
of two or more types. The first two, however, are most generally used.
The largest "dish" is the one just completed and now being tested at Jodrell
Bank in England. This has a diameter of 250 feet, and is steerable, which will allow
it to cover all of the visible sky. It will complement the fixed 220-footer in operation
there for many years. Work has also begun on a 140-foot steerable dish at Green
Bank, West Virginia, which will be the largest of its type in the United States.
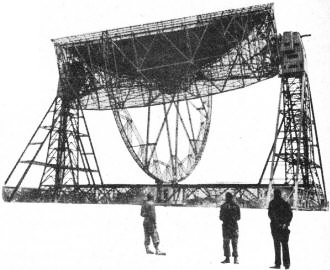
The largest parabolic radio. telescope is at Jodrell Bank, England;
a movable dish, it is a 250-foot monster which has also been used in tracking the
Russian Sputniks.

Photo of Jupiter, showing the huge "Red Spot" in the upper left-hand
segment; this largest of planets is a radio source as well.
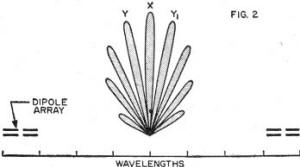
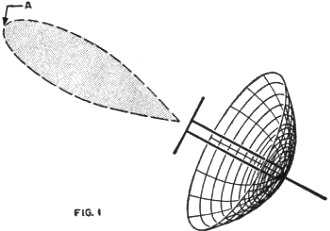
How a Radio Telescope Works
Another new scope is under construction at the University of Michigan - this
85-foot dish will be completed in time to aid the International Geophysical Year
effort. Recently completed was a long-range radar station at Lexington, Mass. Built
by the Lincoln Laboratory, this radar has been used successfully to track the Sputniks.
It is also being used to check the radio effects of meteors and the aurora.
One of the largest interferometers is near Sydney, Australia. This consists of
an array of dipoles 1500 feet long.
Essentially, the job of the radio telescope is to focus the radio waves it receives
and feed them into a sensitive receiver. This is analogous to the action of the
optical telescope. The parabolic dish, either solid or made of wire screen or mesh,
reflects incoming radio waves to a focal point, where they are picked up by a rod
or dipole and fed to a receiver. The signal is amplified, then sent to a mechanical
recorder, usually a pen tracing the signal on graph paper.
The interferometer, on the other hand, works in a different manner. The typical
telescope of this sort consists of a flat array of dipoles. When they face directly
toward the emission source, the wavefront reaches all the dipoles at the same time.
This is shown as a signal of maximum strength. When the signal comes in at a slight
angle, it reaches one dipole earlier than the next, and the interference of the
out-of-phase waves cuts the signal strength. To improve resolution, interferometers
are built with a second array of dipoles arranged at right angles to the first array.
Where the two signals of maximum strength intersect, they produce a "pencil" beam
which has the resolution of a huge parabolic antenna.
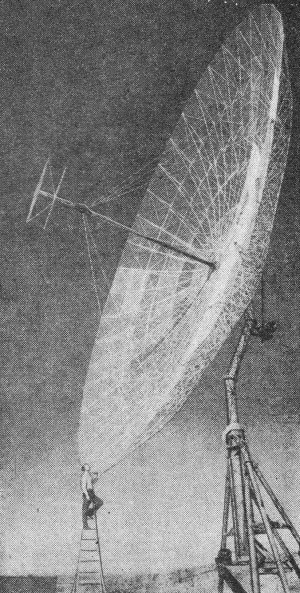
The 61-foot movable parabolic antenna at Stanford Research Institute
in Menlo Park, California.
Diffuse Definition
Radio waves from space are relatively unaffected by daylight, cloud or fog, which
is a tremendous advantage over light waves for observation, but their long wavelength
compared to light makes it difficult to gain good resolution. The beam width depends
on the ratio of the wavelength to the diameter of the telescope. Therefore, in order
to gain the resolution of even a small optical telescope, the antenna of a radioscope
would have to be thousands of miles long. For that reason, radioscopes are able
to define a radio source only diffusely, causing the observers to concentrate on
the shorter wavelength. But as the larger scopes are built, they will be able to
push their studies up into the longer wavelengths. There is no doubt that even more
and possibly greater surprises are awaiting them in this region.
With the earth trembling on the threshold of space, it would not be out of place
to predict that man may one day soon construct even larger radio telescopes between
orbiting space stations, or even on the moon itself. It is impossible to guess what
tremendous discoveries there will be, but one thing is certain - radio astronomy
will some day rank with optical astronomy as one of the most important of sciences.
Indeed, it may even outgrow its older brother in unlocking the secrets of the universe.
It won't be long now!
Radio telescopes are tuned to receive certain radio frequencies and indicate
the direction from which they come. The two most common, the parabolic reflector
(dish) and the interferometer, use two different methods to gain the same end. Note
that in the dish (Fig. 1) there is a single response pattern, a fairly wide
one. The dish is used just like an optical telescope: it "focuses" on a point as
closely as possible - by using the maximum response point "A" on the radio source.
On the other hand, the interferometer (Fig. 2) responds with a series of lobes
as the angle of observation of the radio source changes, alternately reinforcing
and canceling. Using the angles between these peaks, such as between "X" and "Y,"
the position of the radio source can be calculated with a somewhat better degree
of accuracy.
Posted September 20, 2022 (updated from original post
on 10/31/2013)
|